I am extremely excited to share a guest article from Zac Robinson and Josh Pelland. Zac and Josh are both masters students at Florida Atlantic University, and are coaches and co-owners of Data Driven Strength . I have really enjoyed chatting with, and bouncing ideas off of both of them over the past year or so as we share a lot of similar interests and has helped me refine my own thoughts and understanding of the training process. They crushed this article. I got a lot out of reading this and I believe it brings a ton of value and compelling points to the discussion of optimizing strength outcomes in the short to moderate term. Enjoy!
-Brian
By: Zac Robinson and Josh Pelland
Based on the principle of specificity, most would assume that training to or close to failure (0-3 RIR) is the way to go for maximal strength. However, the longitudinal data do not align with this notion. In this article, we will explore how the force-velocity curve and intraset fatigue influence training specificity for maximal strength.
5,300 words; ~17 minute read
Key Takeaways:
- We make the argument that the force production of a repetition is a vital and often underemphasized aspect to training specificity for maximal strength. Training with heavy loads and low intraset fatigue/velocity loss results in force production the most specific to a 1RM.
- For short to moderate term strength gains, performing sets to or close to failure doesn’t offer an additional training effect but can cause other detrimental changes. We also discuss the interplay between muscle growth and proximity to failure for long term strength gains.
- The available research suggests that when training with 65-85% of 1RM, staying far from failure allows for the accumulation of repetitions that provide the majority of the strength stimulus. This means that sets can be terminated before large decreases in bar velocity occur (roughly 10-20% velocity loss) while still maximizing the strength stimulus. We also recommend incorporating regular heavy top sets (>85% of 1RM) for skill practice at velocities similar to a 1RM.
Introduction
For maximal strength, specificity is often touted as the king of training principles. The principle of specificity suggests that you should perform the task you want to improve in the way it will be assessed. For strength, this is primarily discussed in the context of load (% of 1RM) and exercise selection. Consequently, regular exposures to heavy loads using the exact exercises you want to improve are recommended by most coaches.
Another aspect of specificity is a set’s proximity to failure. This is often quantified as the number of repetitions in reserve (RIR) when a set is terminated. By definition, a 1RM is a maximal effort, so this would require 0 RIR. Thus, the principle of specificity seems to suggest that training close to 0 RIR is the way to go for strength. However, a growing body of evidence suggests that training far from failure is just as effective for strength gains and could offer additional theoretical advantages. In this article, we will examine this apparent contradiction and offer practical takeaways based on the existing evidence. Before we review the longitudinal research, let’s rethink how proximity to failure influences specificity for maximal strength. We’ll start by exploring the force-velocity curve.
Specificity and the Force-Velocity Curve
A foundational concept in exercise science is the inverse relationship between the force and velocity of a muscular contraction (Figure 1). To illustrate this relationship, let’s compare a vertical jump and a 1RM squat. Due to the high velocity in which a vertical jump is performed, there is not enough time to form a large number of actin-myosin cross-bridges. The fewer the number of cross-bridges generating force, the less total force production. In contrast, a 1RM squat is performed very slowly, allowing sufficient time for more actin-myosin cross-bridges to form. This leads to an increased number of power strokes from myosin motors; thus, peak force production reaches a much higher level than in a vertical jump. This concept comes intuitively to many lifters who know it’s impossible to use all of their force to lift against an empty barbell.
Importantly, in the cases of a vertical jump and a 1RM squat, we are comparing the force and velocity characteristics of two isolated contractions. Said another way, the vertical jump and 1RM squat are occurring in a vacuum where the individual is completely recovered for the performance of each task. As we introduce multiple repetitions and thus the accumulation of fatigue within a set, things get more complicated.
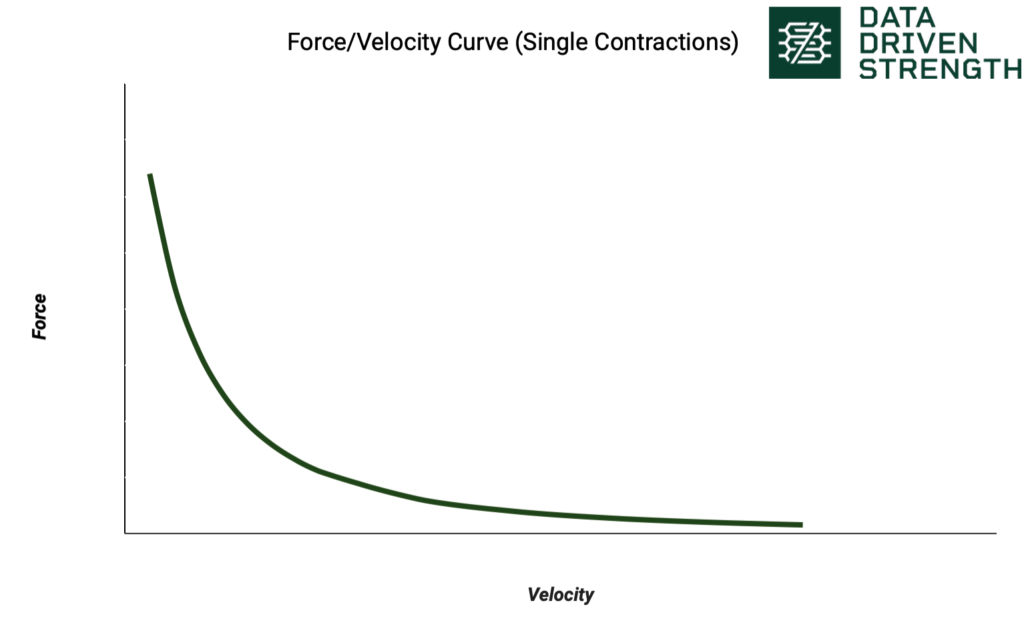
Over the course of a multi-rep set, fatigue accumulates and bar velocity decreases as an individual gets closer to failure. If only considering the force-velocity curve in Figure 1, one may come to the conclusion that force production increases throughout the set. Intuitively, we know this isn’t the case; you don’t get stronger as a set goes on. In reality, as bar velocity decreases throughout a set, there is a concurrent drop in force production (Figure 2). Thus, the force-velocity curve becomes misleading when intraset fatigue is introduced.
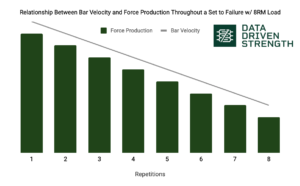
Both a 1RM and a rep at the end of a multi-rep set are performed at a slow velocity for their respective loads, but this does not mean they are both performed with high levels of force. This disconnect is extremely important to consider when discussing specificity for 1RM strength.
As an aside before we move on, it is important to emphasize that we are discussing the total force production of a muscle and not the force production of individual muscle fibers. These values do not necessarily correlate and oftentimes diverge when intraset fatigue is introduced. For example, at the start of a set, all muscle fibers can produce force and thus the muscle’s total force production is maximized. As intraset fatigue accumulates, the most fatigable muscle fibers decrease their force production from the time their parent motor unit is recruited with a near maximal firing rate. As this process continues, there is a gradually smaller pool of muscle fibers responsible for lifting the load. This effectively increases the force produced by individual muscle fibers while simultaneously decreasing the muscle’s total force production.
One may object to the above explanation and cite the All-or-None principle of motor units; that is, when intraset fatigue leads to the recruitment of a motor unit, all of the muscle fibers within it contract. While true, it is important to note that the muscle fiber types within a motor unit are likely not completely uniform. This may be in part due to muscle fiber types actually existing on a spectrum rather than in distinct groups of type I, IIA, and IIX as older research suggests. If this is the case, the most fatigable muscle fibers within a motor unit will “drop out,” increasing the required force production of the other muscle fibers in the motor unit. Keep in mind that the larger the motor unit that innervates a group of muscle fibers, the greater the propensity for muscle growth, the greater the contribution to total force production (due to their large size), and the greater the susceptibility to fatigue. Thus, if our speculations are correct, these muscle fibers that are the most important for maximal strength will produce very little force as the set approaches failure. This may explain why training close to failure makes it nearly impossible to maintain performance across multiple sets. If a set is taken close enough to failure that the most fatigable fibers must “drop out,” their susceptibility to fatigue may lead them to be unable to recover fully for the remaining sets, limiting the total mechanical tension they can experience.
To be clear, there is limited direct evidence on this concept, so please take this explanation with a grain of salt as we could be totally off base. However, this may partly explain why it is appropriate to focus on a muscle’s total force production and not the force production of an individual muscle fiber when discussing specificity for strength. With this in mind, let’s dive a bit deeper into force production and its importance for specificity.
Force Production, Why It’s Important, and How We Maximize It
A 1RM is the ultimate test of force production and encompasses many factors. Some may assume that neuromuscular factors such as motor unit synchronization and intermuscular coordination are independent of force production. However, these factors are a means to the end result of a greater ability to produce force. Due to its central role, we will focus on force production rather than the mechanistic inputs that determine it.
Based on the principle of specificity, it makes sense to train in a way that mimics the high force production in a 1RM. There are two primary variables influencing the force production of a set: load (% of 1RM) and intraset fatigue.
The influence of load on force production is relatively straightforward: higher loads mean higher peak force production. This higher force production likely plays a role in the consistent finding that high load training leads to greater gains in 1RM strength than low load training. You could take this argument to its end and conclude that training with only heavy singles is the best way to train for strength. In fact, this has been supported in the literature to be effective. This reasoning leads us to believe that regular exposures to heavy loads (>85% of 1RM) are a crucial part of training the skill of a 1RM. However, while training with only heavy loads is effective, there’s good reason to believe training in this way would leave long term strength gains on the table.
The primary reason training with only heavy loads (>85% of 1RM) is likely suboptimal in the long term is the consideration of muscle growth. In short, a muscle’s force production capacity is ultimately constrained by its size. Since volume and muscle growth follow a dose response relationship, sufficient volume should be accumulated. Practically, when only using heavy loads (>85% of 1RM), more sets are needed to maximize muscle growth, making this approach challenging and inefficient. A common solution to this problem is to include “volume work” with 65-85% of 1RM to allow for more repetitions per set and thus more efficient muscle growth. Additionally, this allows for more opportunities to practice the skill. These factors may explain why strength gains also follow dose response relationship with volume, albeit to a lesser extent than muscle growth.
Due to this drop in load for “volume work,” force production will inevitably decrease. How do we make this training the most specific to a 1RM given these constraints? This is the question we look to answer with the rest of this article.
This is where the influence of intraset fatigue on force production becomes important. As mentioned earlier, as intraset fatigue accumulates, both bar velocity and force production decrease (Figure 2). Therefore, in regard to force production, it is the repetitions early in a set that are most specific to a 1RM. This suggests that we should minimize decreases in bar velocity throughout a set in order to focus on the repetitions that really “count” for strength. An additional benefit is that we are not wasting recovery capacity on repetitions in which our muscles produce significantly lower force.
There are potentially large benefits of minimizing the recovery cost of training to or close to failure (0-3 RIR). Most importantly, training to or close to failure causes more muscle damage than training far from failure. This muscle damage can decrease performance for up to 48 hours, which could drastically reduce the load and volume utilized on subsequent training days. Additionally, this may require a reduced training frequency. This is undesirable as Stronger by Science’s in-house meta analysis demonstrates that higher frequencies are generally superior for strength gains. Moreover, muscle damage attenuates motor learning and skill acquisition. As we’ve mentioned, a 1RM can be viewed as a skill, and excessive muscle damage impairs your ability to practice the motor skill with precision. Finally, training to or close to failure could provoke other detrimental neuromuscular changes for 1RM strength. For example, gains in vertical jump height, which can be used as a proxy for rate of force development, seem to be attenuated when training close to failure. While rate of force development is not the primary concern for powerlifters, there is an argument to be made for its utility in overcoming a sticking point in a lift.
One may argue that some research suggests training to or close to failure results in more muscle growth than training farther from failure. This is a fair point as some intraset fatigue is likely necessary to optimize muscle growth. However, the amount of intraset fatigue needed is less than most originally thought. Overall, the body of evidence suggests that we do not need to train extremely close to failure to induce the majority of muscle growth a set has to offer. Therefore, while training far from failure to maximize force production, we can still cause muscle growth. This is actually great news. We are able to avoid the repetitions late in a set with less force total production and still induce a reasonable growth stimulus. Further, since we’ve avoided the mentioned consequences of training to or close to failure, we can perform more sets, which is generally a good thing for both strength and muscle growth outcomes.
To be clear, the above discussion of proximity to failure and muscle growth is most relevant in the short to moderate term. For example, performing “volume work” farther from failure during the eight weeks leading up to a strength test or competition allows for high force repetitions to be prioritized while providing a sufficient training stimulus to maintain muscle mass. However, when far from testing your strength, it may make sense to train closer to failure to ensure you’re maximizing growth on a per set basis.
Let’s summarize where we’re at so far. When considering force production, repetitions far from failure are more specific for strength than those closer to failure. Moreover, training to or close to failure can result in some serious drawbacks. With this framework in mind, let’s examine the longitudinal research.
Research Examining the Influence of Proximity to Failure on Strength Gains
Most of the research up to 2015 on this topic can be summarized by a meta-analysis (and an erratum) by Davies and colleagues. They found no difference in strength gains between groups training to and not to failure.
Before we get ahead of ourselves, there is a significant limitation with the literature included in this meta-analysis. Most of the included studies utilized a fixed number of repetitions at a given percentage of 1RM for the non-failure group. This is troublesome as the maximal number of repetitions that can be performed at a given percentage of 1RM varies greatly between individuals. Consider data from Cooke and colleagues which found a range of 6-26 repetitions when individuals performed a set to failure with 70% of 1RM on the squat. This means that when prescribing 3 sets of 8 repetitions at 70% of 1RM, some individuals would fail while some would have over 15 repetitions in reserve. Consequently, we can’t confidently quantify the proximity to failure at which the non-failure groups were training.
Thankfully, this area of research has continued to develop over the last few years, and multiple studies have addressed this limitation. Many of these studies have better controlled proximity to failure via a method called percentage velocity loss. In essence, this allows all subjects to use the same percentage of 1RM and perform repetitions until bar velocity drops a predetermined percentage from the fastest rep (usually the first or second rep). This method isn’t perfect either, but it is certainly an improvement. To be clear, some of the studies included in our coming analysis either controlled proximity to failure by other means or didn’t control it at all. These latter studies thus have the same limitation as the studies from the Davies meta-analysis, but we have included them as they still offer valuable information.
To the best of our knowledge, the following is a collection of all the research examining proximity to failure’s influence on strength gains since the publication of the Davies meta-analysis in 2015. We chose to include a study from 2013 by Oliver and colleagues as it was excluded from the 2015 meta-analysis but has important insights.
*If you are primarily concerned with the conclusions and practical takeaways, feel free to skip these study reviews. We highlight all of the main points in the sections to follow.*
Click here for a detailed breakdown of the included studies.
Research Recap
Let’s take a step back and get the lay of the land. Out of the 15 studies reviewed, all but two found no significant between-group differences in strength gains. The other two found significantly greater strength gains in the group training farther from failure. When compiling all of these studies, groups training far from failure slightly outperform groups training to or close to failure (15.6% vs 14.0% average strength gain; Figure 3). These findings fit in well with the results of the Davies meta-analysis.
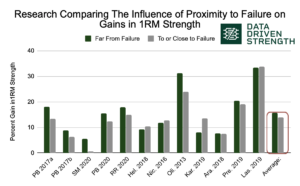
Set Equated Studies
Remember, based on force production, it is the first few repetitions in a set that are the most specific to maximal strength. In set equated studies (e.g., 3 sets of 10 with 75% of 1RM vs. 3 sets of 5 with 75% of 1RM), both groups are exposed to these first repetitions, so we’d expect similar strength gains. When only considering the studies that equated sets (Pareja-Blanco 2017a, Pareja-Blanco 2017b, Helms 2018, Lasevicius 2019, Caroll 2019, Galiano 2020, Sanchez-Moreno 2020, Pareja-Blanco 2020, and Rodriguez-Rosell 2020), the groups training farther from failure slightly outperform groups training to or close to failure (15.5% vs 13.1% average strength gain).
Total Repetition Equated Studies
In total repetition equated studies (e.g., 3 sets of 10 with 75% of 1RM vs. 6 sets of 5 with 75% of 1RM), the results are less straightforward. Theoretically, the groups splitting their volume into more sets should be performing more repetitions with high force production, leading to greater strength gains. However, when only considering the studies that equated total repetitions (Nicholson 2016, Helms 2018, Arazi 2018, Oliver 2018, Prestes 2019, and Karsten 2019), there is no practical difference in average strength gain (14.7% vs. 14.5%). This was a surprising finding, so we dug a bit deeper into these repetition equated studies.
Right off the bat, the study by Helms and colleagues used a different design than the others – they equated both repetitions and sets. Although one group used slightly less load, they still performed the same number of repetitions with high force production, so the equal strength gains between groups are expected. The Arazi study presents some practical issues in its application. In the last two weeks, both groups trained with 30-55% of 1RM. There were no differences in strength gains in this study, but this tapering strategy may have washed out any differences.
The study by Prestes and colleagues had a unique design – they compared a traditional multi-set group to a rest-pause training group. The traditional multi-set group trained with 80% of 1RM for 3 sets of 6 repetitions and 2 minute rest between sets. The rest-pause group also trained with 80% of 1RM but performed sets to failure with 20 seconds rest between sets until they completed the same total of 18 repetitions. It’s likely that the rest-pause group performed more sets, and as mentioned, this would usually be an advantage. However, because the rest-pause group used extremely short rest periods and sets were taken to failure, this advantage likely disappeared due to fatigue carrying over from previous sets, ultimately shifting the advantage to the traditional multi-set group. Despite this, no difference in bench press, leg press, or bicep curl strength gains were found. However, this may be explained by an inherent advantage in load progression that groups training to failure receive. The study did not include any details on how training progressed for either group, but the failure training the rest-pause group used may have been used as a proxy of progression. On the other hand, there was no way to know whether the load used for the 3 sets of 6 repetitions in the traditional multi-set group became way too light. If the traditional multi-set group never progressed load, they would have used a gradually more submaximal load as they got stronger, giving an edge to the rest-pause group. Lastly, it’s important to consider that the rest-pause group generally saw greater muscle growth than the traditional multi-set group. This may indicate that the traditional multi-set group made up for this difference in muscle growth by performing a greater number of repetitions with high force production. All in all, this study is interesting to ponder, but it’s hard to tell exactly how it fits into the big picture of how proximity to failure influences strength gains.
This brings us to three studies that are designed in a way that we would expect to see greater strength gains in the groups that were performing more sets: Oliver, Nicholson, and Karsten. In addition to equating total repetitions, these studies equated load, so one group performed more sets and finished their sets farther from failure.
The study by Oliver and colleagues provides an example of how we would expect this design to play out based on our hypothesis. One group performed 4 sets of 10 with 65-75% of 1RM and the other group performed 8 sets of 5 with the same load. The group performing 8 sets of 5 accumulated more repetitions with high force production, so this group experiencing significantly greater 1RM strength gains in the squat was expected. While changes in bench press 1RM were not statistically different, this leaned in favor of the 8 sets of 5 group as well (13.6% vs. 8.7%).
The study by Nicholson and colleagues offers a second opportunity to examine this concept. Although this study had four groups, there are two groups we are primarily concerned with. The strength group performed 4 sets of 6 repetitions with 85% of 1RM and the cluster-1 group also performed 4 sets of 6 repetitions with 85% of 1RM but took a 25 second rest between each repetition. This allowed the cluster-1 group to accumulate less intraset fatigue and thus stay farther from failure. This was confirmed by the reported average velocity loss per set – the strength group averaged 40.3% velocity loss and the cluster-1 group averaged 8.7% velocity loss. Based on the cluster-1 group performing their sets with lower velocity loss and thus higher force production, we’d expect the cluster-1 group to have experienced more strength gains, but no difference between groups was found. Honestly, we’re scratching our heads with this one. However, when thinking through this study design, it’s likely that the cluster-1 group had to unrack and rerack the bar for every repetition. Although this isn’t stated in the study, we assume that the bar had to be unloaded somehow. It’s possible that the cluster-1 group performed 24 unracks, walkouts, and reracks with 85% of 1RM while the strength group performed only 4. We’d expect the fatigue from these unracks to limit force production, but the reported velocity losses does not indicate this was the case. We’re not sure what to make of this, but it’s something to consider when conceptualizing these results.
Perhaps the most important study to dissect is by Karsten and colleagues. At face value, it is almost a direct clone of the Oliver study as one group performed 4 sets of 10 with 75% of 1RM and the other performed 8 sets of 5 with the same load. The researchers intended to have the 4 sets of 10 group train to failure and the 8 sets of 5 group train with about 5 RIR. However, there’s a key difference in how load was manipulated in the 8 sets of 5 group. Unlike the Oliver study, the 8 sets of 5 group autoregulated load based on the OMNI-RES scale. The OMNI-RES scale is a general rating of exertion and thus does not have an objective anchor like the RIR scale. This results in a wide range of interpretations across individuals.
In the Karsten study, the group performing 8 sets of 5 was not allowed to pass a rating of 7 on the OMNI-RES scale and dropped the load if they did. Other research indicates that individuals reach an average of 7.64 and 6.80 OMNI-RES rating with 70-80% of 1RM after only a 10% velocity loss on the squat and bench press, respectively. To get all the way to failure with the same loads, there is an average of 50.9% and 69.4% velocity loss on the squat and bench press, respectively. This means that only about 15-20% of the possible repetitions were completed before an individual rated the set around a 7 on the OMNI-RES scale. Practically, this means that instead of using the intended load of 75% of 1RM to elicit 5 RIR, load was likely dropped to elicit something closer to 8-9 RIR. While the 8 sets of 5 group was likely at a force production advantage in terms of intraset fatigue, they were likely at a disadvantage in terms of load. This potentially resulted in the same total force production via two different routes, explaining why this study did not find different strength gains between groups.
How Far From Failure is the Sweet Spot?
Although the presented evidence isn’t completely unanimous (namely, Nicholson), we can tentatively construct a model that helps to explain our overall findings. To revisit the theme of this article: since the first few repetitions in a set are the ones with the highest force production, they presumably provide the majority of the strength stimulus. As bar velocity and force production decrease concurrently throughout a set, the repetitions offer less and less of a strength stimulus. Also, these repetitions induce more fatigue. So, throughout a set, the strength stimulus per rep decreases while the fatigue per rep rises. Due to this, it may be best to prioritize bar velocity for the given load as this is essentially a proxy for force production.
Three of the reviewed studies (Pareja-Blanco 2017a, Pareja-Blanco 2017b, and Pareja-Blanco 2020) used an equated sets design with differing velocity losses between groups. Further, all three used the same exercise (smith machine squat), same loads (70-85% of 1RM), and 4 minute rest periods. When compiling the results of these studies, a 10-20% velocity loss per set (which roughly correlates to as high as 10 RIR, depending on the load) seems to be the point at which more velocity loss does not lead to greater strength gains in the 6-8 week time frame of these studies (Figure 4). While these findings are expected based on the rest of the literature, we don’t intend for this to be a set in stone recommendation as we would like to see these findings replicated beyond these three studies.
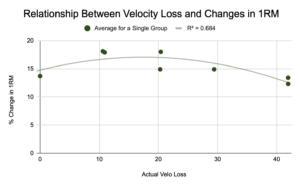
It is important to emphasize that we cannot make conclusions about exposures to heavy loads (>85% of 1RM) as none of the reviewed studies included these. However, there is good reason to believe that including exposures to loads >85% of 1RM will better develop technical proficiency at the slow velocities that are inevitable during a 1RM attempt. With that caveat, let’s wrap up by discussing how we can practically apply all of these ideas.
Conclusion
Maximizing exposure to high force production is an important aspect to specificity for strength gains. While using greater loads increases force production, it is not practical to accumulate all of the required volume with very high loads. When load is dropped for “volume work,” minimizing intraset fatigue by avoiding close proximities to failure provides the greatest return on investment. This is because the first few repetitions will have the highest total force production at a given load. Training to or close to failure includes these repetitions, but many repetitions are also performed where force production has dropped off considerably. Further, there is disproportionately greater fatigue when taking sets to or close to failure. Combine “volume work” far from failure with regular exposures to heavy loads (>85% of 1RM), and you will also accumulate the skill practice and confidence necessary for 1RM performance.
To be clear, completing the “volume work” far from failure is only supported to maximize strength gains in the short to moderate term (6-12 weeks). When training for long term strength gains, muscle growth will become more of a focus. It’s probably a good idea to perform sets closer to failure when muscle growth is the primary goal as these sets are likely more efficient for muscle growth on a per set basis.
As a final point, we want to emphasize the importance of performing all repetitions with maximal concentric velocity. Greater acceleration of a given mass means greater force production. As we’ve argued in this article, a higher force production is more specific to a 1RM. This aligns with research finding superior strength gains in subjects moving all repetitions with maximal concentric velocity compared to subjects performing repetitions with a deliberately slower cadence.
Practical Applications
Load:
- Include exposures to heavy loads (>85% of 1RM) to practice technical proficiency at slow velocities and achieve peak levels of force production.
- Include sets at 65-85% of 1RM to more efficiently accumulate volume.
Proximity to Failure:
- Heavy sets will inevitably be closer to failure as they approach 100% of 1RM.
- Minimize per set velocity loss on “volume work.” Some evidence suggests that the per set strength stimulus is maximized around 10-20% velocity loss (which roughly correlates to as high as 10 RIR depending on the load).
- Training this far from failure is really hard to gauge, so here are a couple ideas for doing so successfully:
- Perform a load/rep combo that will be far from failure for almost everyone (e.g., 75% of 1RM for sets of 4).
- Perform one set with as many repetitions as it takes to get to 1-2 RIR with a given percentage of 1RM. Then, drop off the number of repetitions per set that gets you to the desired RIR (e.g., 80% of 1RM for 8 repetitions with 2 RIR, then perform more sets with the same load with only 4 repetitions per set).
- Sets in which muscle growth is the primary goal should be performed closer to failure than the above recommendations.
Tempo:
- All repetitions should be performed with maximal concentric velocity. This maximizes force production at a given load.
Volume and Frequency:
- An advantage of training far from failure is less fatigue and muscle damage per set. Due to this, you may be able to complete more weekly sets and use higher frequencies.
Rest Periods:
- Rest periods should be long enough to avoid a drastic decrease in bar velocity from set to set. The length to accomplish this can be autoregulated to manage total training time.
Acknowledgements
We would like to thank John Hanley and the Exodus Strength Forum for sparking our interest on this topic and providing excellent discussion that has helped form our thoughts.